About 40% of the 10.3 million visits to National Health Service (NHS) emergency departments in England in 2009/10 are recorded as ending with the patient needing advice and no specifc treatment (HESonline, 2011). It has previously been suggested that these needs could be met in the community through the delivery of urgent (or pre-hospital) care (Department of Health, 2005) by emergency care practitioners (ECPs) (Department of Health, 2004).
An ECP may encounter a wide range of presenting complaints in varying situations requiring different equipment and consumables. Reynolds (2008) found that ECPs made individual decisions about both the contents and transportation methods of their kit, including using bag systems ranging from rucksack to ice cream tubs. A previous project, ‘Smart Pods’, explored the technical requirements for ECP work by collecting data about pre-hospital care using stakeholder workshops, portable technology audits, treatment observations and design decision groups with 125 staff and 88 patients (Hignett et al, 2010; 2011). The results were design specifcations for modular treatment units as personal kit, assessment pods and packs and a new design for the clinical work space (Hignett et al, 2010; 2011). This project aimed to take these outputs and develop them into a Community Urgent Response Environment (CURE) as:
This paper describes the design, testing and evaluation cycles to develop the CURE technology system through a knowledge transfer collaboration of two universities (Loughborough University and University of the West of England), two manufacturers (Openhouse Products and WAS Vehicles (UK) and the National Health Service (Great Western Ambulance Service NHS Trust, GWAS).
Method
The project protocol used an iterative prototyping approach (Figure 1).

For the frst activity the research team reviewed the outputs from Smart Pods (Hignett et al, 2010; 2011) and transformed them into functional design requirements as prototype pod/pack 1.0 (Table 1). This resulted in a core assessment pod (to be used for every patient) and supplementary pods for specifc presenting complaints. Individual pods had detailed content design, for example the Wound Care Pod has four internal packs to group functional equipment and consumables.
The initial pod/pack prototype concept (1.0) was built by one of the manufacturing research team members (Openhouse Products) as pod/pack prototype 1.1 for user trial 1 (Figure 2). After trial 1 the results were reviewed and incorporated in the iterative prototyping process as pod/pack prototype 1.2, with the final version (pod/pack prototype 1.3) built after trial 2 (Figure 3).


The trials were carried out in simulated settings to represent a bedroom (confined household environment), and a public location in trial 1. For trial 2, the vehicle (mobile treatment unit) interface was built by the second manufacturing research team member (WAS Vehicles). Five paper-based prototypes were reviewed and re-designed before the full scale mock-up was constructed (vehicle prototype 1.5, Figure 4).
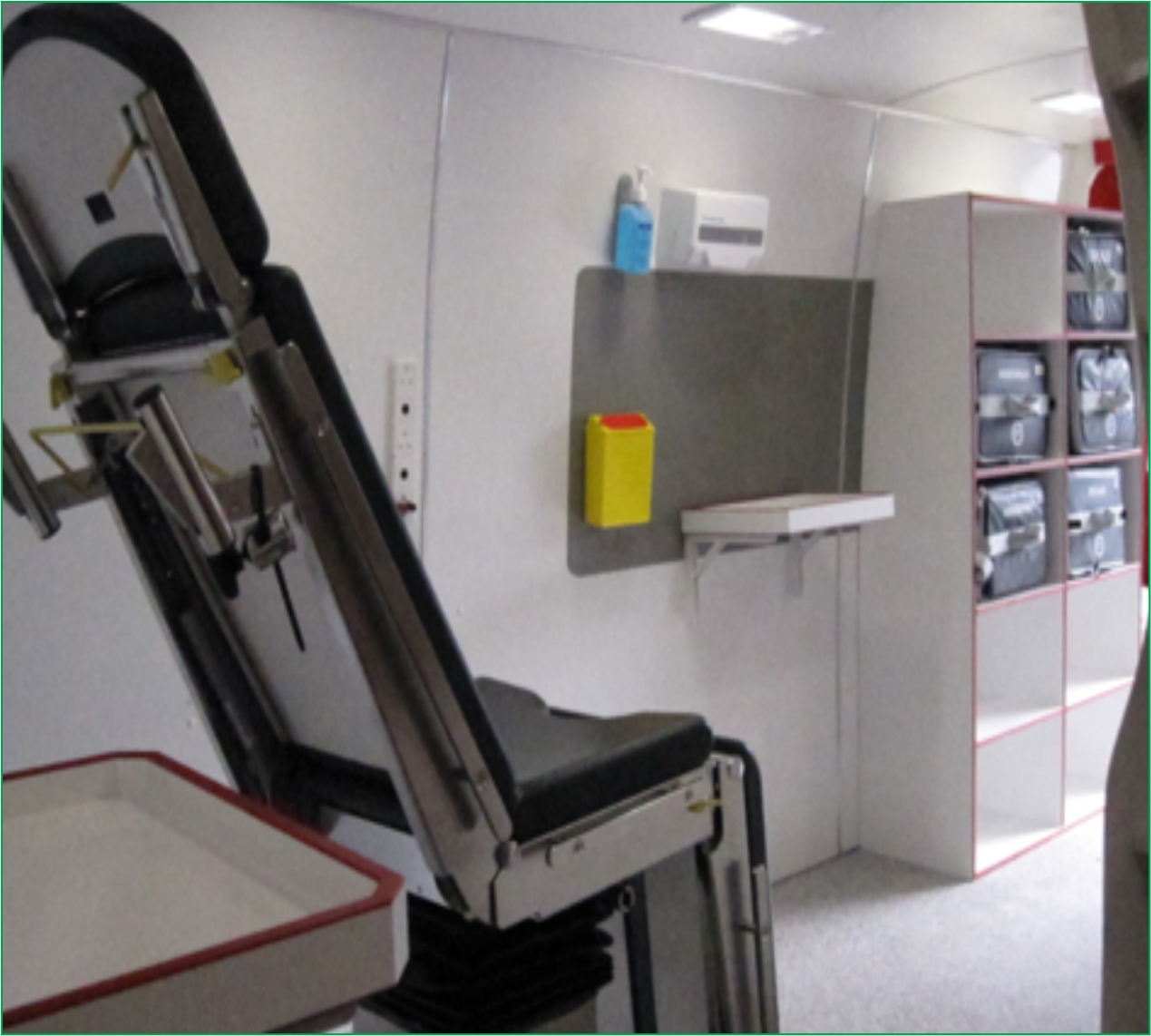
Participants
Twelve ECPs participated in the two user trials (six participants in each trial). A randomised repeated measures design was used with the condition exposures (presenting complaints) split across the scenarios.
Virzi (1992) and Lewis (1994) suggest that five participants should identify 80% of the usability issues.
One way to enhance the discovery of usability issues and maximise the likelihood of achieving problem identifcation is to improve participants’ knowledge (Lewis, 1994; Kanis, 2011). This was achieved by providing detailed information to the participants before and during the trials and ensuring repeated exposures to the tasks and equipment through the design of the scenarios.
Scenarios
Clinical scenarios were selected from a review of patient notes (DC) and developed (JB, DC, MF, SH and NM) to ensure that participants would experience a wide range of equipment and consumables from the CURE pods and packs. Four scenarios were used to test the systems; head injury, paediatric febrile convulsion, chest pain / respiratory and collapse from an unknown cause (Table 2). The clinical information was delivered as a simple radio communication template (pre-arrival at scene) and as a set of test results as appropriate during the scenario to respond to the clinical tasks undertaken by each participant.
NHS ethical (NHS REC 10/H0406/81) and research governance approvals were granted. Written informed consent was obtained from each participant.
Data collection
Each trial was recorded simultaneously by up to three digital video cameras. The data files were coded to maintain anonymity. At the end of each scenario (and each trial) a debriefing interview was used to discuss key issues with the participants who were audio–recorded.
For user trial 1 additional data were collected using post-trial interviews (audio–recorded) by asking the ECP to give a commentary (narrate) the last two scenarios from their trial to describe their interaction with the work station. They were asked to explain their chosen methods of use and give their opinion about the functionality of the pods and packs in relation to their work. Where appropriate, prompts were used to encourage the participants to verbalise their thoughts.
Results
Twenty-four data sets were collected in trial 1 from six ECPs each completing four scenarios. The 72 video datasets (three camera positions) were individually scrutinised for actions and behaviours relating to the use of the pods/packs to capture the main activities. These data were amalgamated with the 24 post-scenario de-briefing interviews and verbatim transcriptions from the 12 post-trial interviews. For trial 2, 23 data sets were collected with five ECPs completing four scenarios and one ECP only completing three scenarios due to time constraints. The data from the 69 videos were scrutinise and the results amalgamated with the de-briefng interviews (n=23).
The analysis involved iterative steps to ensure that all data were accounted for and included in the final results. The first stage of data analysis was the identification of preliminary codes (MF). This was followed by a detailed thematic analysis (Robson, 2011) by importing all the data from both trials into NVivo9 (Richards and Morse, 2007). The scope of leftics (themes) and their relationships are shown in Figure 5.

The emergent themes were compared and contrasted across the datasets and between the two user trials to clarify concepts and explore the interpretations for similarities and differences (Table 3).
As part of the iterative prototyping process, the design changes from trial 1 were reviewed (Figure 1) and where possible incorporated in the subsequent prototype (pod/pack 1.2). For example:
Some of the feedback could not be addressed within this project but shall be taken forward in future research.
Discussion
While the ECP role has developed in different ways across the UK (Mason et al, 2012), the patient group they are intended to serve is well-defned. Although previous research had successfully developed the design specification for the CURE technology (Hignett et al, 2010; 2011), it was found that the participating ECPs also worked as solo rapid responders. This was considered to be a limitation of the research protocol, as some participants had difficulty differentiating between their equipment and consumable requirements for emergency and urgent care responses. This was less of a limitation for the mobile treatment unit, where it was found that the ECP role had potential to be enhanced (including the delivery of a wider range of services) by an improved environment for care.
Although the project focused on the design, testing and evaluation of the 2 components of CURE, some of the de-brief interviews raised operational issues. These were not part of the project's scope but included: ‘just-in-time’ equipment supply and ‘make ready' teams (including carriage of spare pods/packs); standardised labelling for dressing supplies; inaccuracies in service communications with respect to selecting which pods/packs to take to the scene; access to the mobile treatment unit for patients with mobility limitations (design specification limitation); and a process for the transport of patients to hospital when required. The response from the research team to this last point was that a second vehicle (emergency ambulance or patient transport vehicle) would need to be called for transportation where required.
One of the major design innovations of CURE is the incorporation of a work surface into the assessment and wound care pods. Some differences in use were recorded and informed the design development from pod/pack prototype 1.1 to 1.3 (Figure 2 and Figure 3). It was felt that the final prototype (1.3) would allow all the observed working methods to be supported (particularly wound care); this will be further evaluated in future research with solo emergency responders. The mobile treatment unit was found to offer considerable benefits for several design features including 360 ° access to patients, separation of clean and dirty equipment and consumables from the layout and storage areas and improved lighting for clinical tasks.
CURE has achieved one of the key design requirement outputs from Smart Pods by creating a small footprint for the portable systems with improved access to equipment and consumables. Many of the participants also commented that the pod/pack systems might lead to improvements in standardisation of equipment and consumables due to the rigidity of the pod envelope (unable to carry additional equipment) and thus also improve storage and transportation by reducing crushing and cluttering.
Conclusion
The final prototypes (pod/pack 1.3 and vehicle 1.6) have potential to support a new way of working in the provision of non-critical, pre-hospital care. In addition to developing the design specifications from Smart Pods through to manufactured project, the user trials also identified possible efficiencies through the use of CURE by providing support for a wider range of assessment, diagnosis and treatment.