Cardiac arrest due to hypothermia is considered a special circumstance (Deakin et al, 2021; Lott et al, 2021). This is on account of hypothermia being neuroprotective in some situations, which may result in improved prognosis following cardiac arrest (Tipton, 2003; Paal et al, 2016; Harris and Jones, 2018; Foggle, 2019; Lott et al, 2021; Association of Ambulance Chief Executives (AACE), 2022). Hence, guidelines recommend prolonged resuscitation attempts for these patients (Deakin et al, 2021; Lott et al, 2021; AACE, 2022). This has led to the teaching mantra ‘you're not dead until you're warm and dead’ (Paal et al, 2016; Foggle, 2019). However, this is only applicable to patients in whom brain cooling has occurred (e.g. hypothermia as primary cause) prior to cardiac arrest (Deakin et al, 2021; Lott et al, 2021; AACE, 2022). It is not applicable to those who have suffered a cardiac arrest and then cooled as a result.
A hypothermic resuscitation strategy involves the use of mechanical chest compression devices, analysis of biomarkers to determine viability, and active external and core rewarming, including the use of extracorporeal life support (ECLS), in specialist centres (Paal et al, 2016; Deakin et al, 2021; Lott et al, 2021). These efforts are invasive, prolonged and require significant resources. From both a service provider and patient perspective, pursuing such a resuscitation strategy should be based on an informed and judicious decision. The crux for responders when deciding on the most appropriate resuscitation strategy is determining:
The immersion and submersion elements of the incident complicate this decision-making as pulseless casualties recovered from water are also typically hypothermic when their temperatures are measured.
This paper presents a short recap of the pathophysiology and aetiology of cooling and hypothermia for immersed and submerged casualties. The aim of presenting this background information is to promote informed decisions about the appropriateness of applying a hypothermic resuscitation strategy to casualties in this sometimes-complicated setting.
Pathophysiology
Hypothermia causes cellular metabolic rate to fall, leading to reduced oxygen demand and a reduction in neural activity (Harris and Jones, 2018). The brain (and other vital organs) then become more resilient to periods of hypoxia (Paal et al, 2016; Harris and Jones, 2018). The result of this neuroprotective effect is improved prognosis in cardiac arrest (Paal et al, 2016). Crucially, this is only applicable where hypothermia has occurred before, or is the primary cause of, the cardiac arrest. This is because the neuroprotective effects of hypothermia have occurred prior to the hypoxic insult. For patients who suffer cardiac arrest and then cool as a result, the hypoxic insult occurs before the initiation of protective mechanisms.
Establishing the likely mechanism of the incident and chronology of events is crucial to determining the appropriateness of initiating prolonged hypothermia-related resuscitation efforts for casualties recovered from the water (Paal et al, 2016). This requires an understanding of cooling aetiology of immersed (airway out of the water) and submerged (airway under water) casualties.
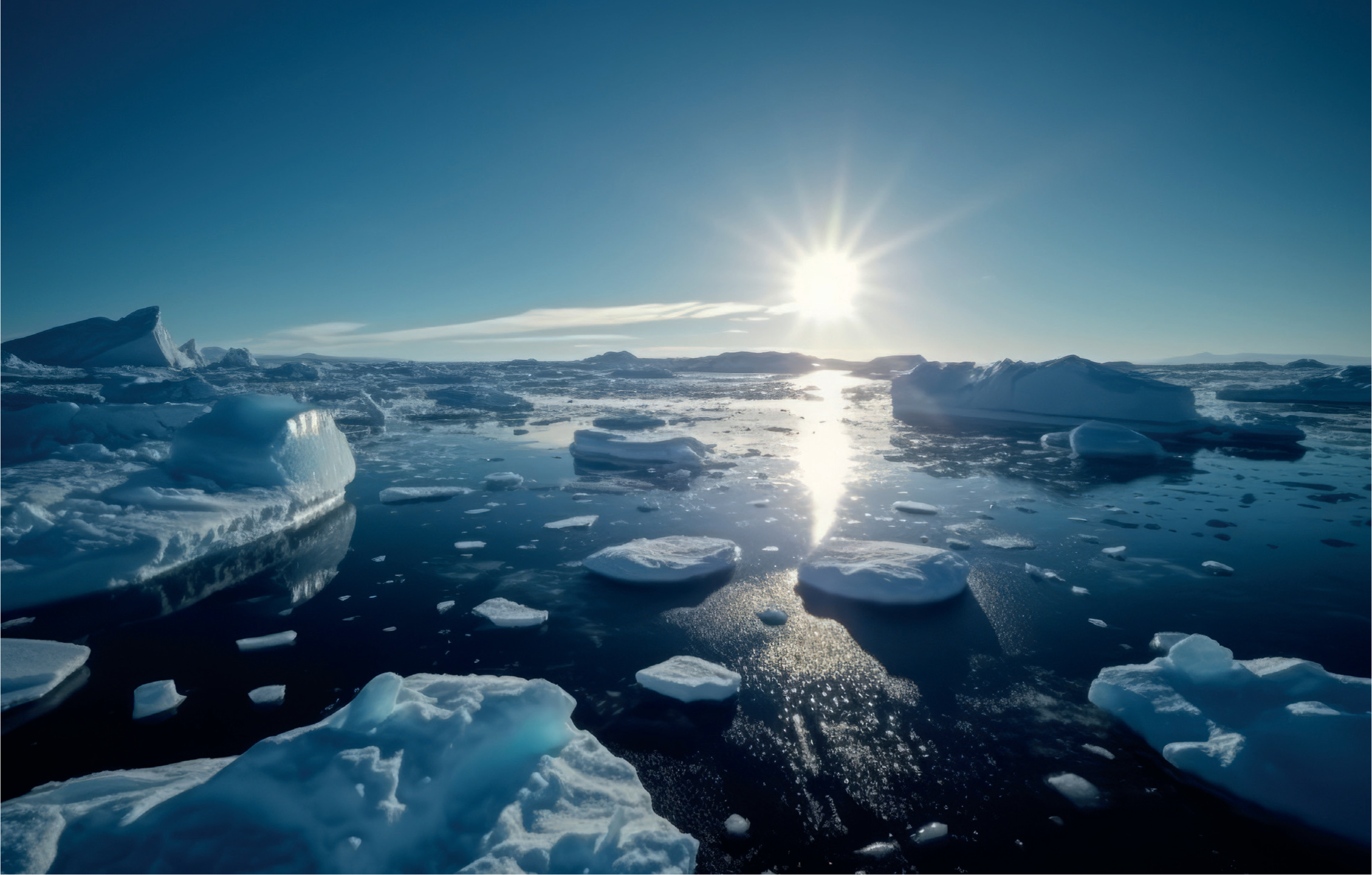
Immersed and submerged casualties: aetiology of cooling
Inducing hypothermic neuroprotective mechanisms to improve prognosis in cardiac arrest requires significant and rapid reductions in brain temperature prior to the loss of oxygen delivery to the brain (Tipton, 2003). This can be generalised as occurring by either external and or internal cooling processes.
External cooling
External cooling involves superficial surface cooling while immersed (Bierens et al, 2016). However, the human body contains too much heat to cool rapidly via surface cooling (Tipton et al, 2022). External cooling resulting in a primary hypothermic cardiac arrest requires the victim to be immersed for several hours in very cold water or exposed to cold weather conditions for a significant period (Tipton et al, 2022). The rate of cooling will vary depending on several factors in addition to the water temperature (Tipton et al, 2022). For example, a lightly clothed smaller victim (child or small adult), with low levels of insulation and higher surface area-to-mass ratios, will cool rapidly compared to the larger victim wearing a survival suit (Tipton et al, 2022).
It is important to note that during the external superficial cooling process, peripheral muscle function is impaired before core temperature is significantly reduced (Bierens et al, 2016; Tipton et al, 2022). Hence, for persons immersed in water, swim failure leading to drowning due to physical incapacitation usually occurs before neuroprotective mechanisms can be initiated (Bierens et al, 2016; Tipton et al, 2022).
A primary hypothermic cardiac arrest caused by immersion in water requires the presence of some form of aid to keep the airway from being submerged (usually a lifejacket), to prevent the victim first succumbing to swim failure and drowning prior to initiation of protective mechanisms (Tipton et al, 2022). Responders should be aware that entrapments in submerged vehicles introduce the possibility that casualties inside the vehicle are considered immersed, and thus subject to cooling before cardiac arrest, if their airway is clear of the water in sub-surface air pockets within the vehicle.
Internal cooling
With internal cooling, breathing and cardiac output continue while the victim is submerged (Tipton, 2003; Tipton and Golden, 2011). Following submersion, the victim will preferentially take water into the oropharynx, then either spit it out or swallow it rather than aspirate into the lungs (Tipton, 2003). This causes cooling of the surrounding structures. The vascular supply to the brain (via the carotid arteries) are in close proximity to these internal tissues; hence, they also cool (Tipton, 2003). This internal cooling effect of the vasculature is accelerated as the same vessels in the neck are also close to the skin surface and, thus, exposed externally to the cold water.
Once the urge to breathe takes over, water is flushed in and out of the lungs (referred to as flushing). This accelerates the cooling of the surrounding organs and vasculature (Tipton, 2003; Tipton and Golden, 2011). In cases where the water temperature is below 15°C, many victims also succumb to cold shock (Tipton, 2003). Cold shock involves an involuntary gasp (2–3 litres) and uncontrollable hyperventilation lasting up to 90 seconds, with increased sympathetic activity. This causes the flushing process to occur almost immediately and increases cardiac output, thus increasing the flow of cooled blood to the brain.
The internal (and external) cooling of the vasculature, accelerated by the flushing process (particularly in the presence of cold shock) results in a very rapid cooling of the blood supply and ultimately the brain itself—thus inducing neuroprotective mechanisms within minutes of becoming submerged (Tipton, 2003; Tipton and Golden, 2011). The brain is then more resilient to the hypoxic insult which follows (Tipton, 2003; Tipton and Golden, 2011; Paal et al, 2016). This phenomenon is termed selective brain cooling (Tipton and Golden, 2011).
Only very cold water is conducive to selective brain cooling as it requires a rapid reduction in the body's core temperature of about 7°C in around 5–10 minutes (Tipton, 2003). Case reports reveal that this rarely occurs in water temperatures above 5°C (Tipton and Golden, 2011). Hence, expert opinion recommends that a water temperature of 6°C is used as the cut-off when considering whether selective brain cooling has occurred (Tipton, 2003; Tipton and Golden, 2011).
Sea temperatures in the UK range between 6–10°C in cold winters to 15–20°C in warm summers. Hence, selective brain cooling is not considered a possibility in UK coastal waters. The temperature of inland waters (lakes, canals, and rivers) in the UK do fall below 6°C. Hence, selective brain cooling should be considered a possibility in these environments—particularly in winter through to early spring, and bodies of water in mountainous regions exposed to extremes of weather.
Discussion
When recovering a pulseless casualty from the water, they are also typically cold. Crucially, this occurs irrespective of the cause of the cardiac arrest. Applying the most appropriate resuscitation strategy (hypothermic versus non-hypothermic) necessitates determining whether hypothermia occurred before, or is, the primary cause of the cardiac arrest, or whether hypothermia occurred because of the cardiac arrest.
It is reasonable to assume that the primary cause of the cardiac arrest could be hypothermia if the patient was submerged or immersed in very cold water (below 6°C); or, if the patient had a prolonged immersion time (hours) in water 6°C or above and their airway was supported above the water to prevent them first succumbing to drowning. If these conditions are met, responders should initiate a hypothermic resuscitation pathway, which includes consideration of transporting the patient to a facility capable of delivering ECLS (Deakin et al, 2021; Lott et al, 2021).
If neither of these conditions are met, it can be assumed that it is likely that the casualty has suffered a non-hypothermic cardiac arrest, e.g. drowned, and then cooled. In these circumstances, prolonged hypothermia-related resuscitation efforts would typically be deemed unnecessary. In such patients, cessation of resuscitation can be considered in the same way as for non-hypothermic patients (AACE, 2022). UK ambulance guidelines account for this by differentiating between drowning and hypothermia; they allow ambulance clinicians to conduct verification of death for patients who have drowned, but not for patients who have suffered a primary hypothermic cardiac arrest (AACE, 2022).
Survivors of hypothermic cardiac arrest following submersion in ice cold water (below 6°C) are usually children (Tipton, 2003). It is hypothesised that this is due to a combination of selective brain cooling being more effective in children and small persons, coupled with the benefit of an increased rate of superficial surface cooling due to their greater surface area-to-weight ratio (habitus) (Tipton, 2003). However, this hypothesis is based merely on a collection of case studies, and extrapolation of physiology and thermodynamic first principles. There is insufficient evidence to allow responders to differentiate between adults and children (or between those with different habitus) when deciding whether a prolonged hypothermic resuscitation strategy is appropriate, after they have been rescued.
Conclusion
Prolonged hypothermia-related resuscitation efforts are applicable to a cold, pulseless casualty recovered from the water when the incident details suggest that they have cooled prior to, or hypothermia is the primary cause of, cardiac arrest. This is typically deemed possible if the water temperature is below 6°C, or they if they have been immersed for a prolonged period (hours) with their airway supported above the water.