Heart block (HB) frequently forms part of the clinical picture of patients suffering acute myocardial infarction (MI) (Gamon et al, 2007). Identifying the type of HB associated with MI is important in determining treatment strategies and predicting the likely clinical course (Fowler, 2002). This is reliant on a sound understanding of relevant anatomy and physiology (Moran and Gunnar, 1975). Although many of the original pathological studies investigating HB were conducted almost half a century ago, their results are still of value in highlighting the role of anatomical and physiological variations in the clinical presentation of HB in the setting of AMI.
Anatomy and physiology
The heartbeat is initiated by an intrinsic electrical system composed of modified myocytes. These myocytes are organised into a group of spontaneously discharging pacemaker cells, situated in the sinoatrial (SA) node, and a series of elongated conduction fibre cells, responsible for propagating electrical impulses throughout the heart (Levick, 2010). The SA node is situated close to the superior vena cava on the posterior wall of the right atrium. It is suggested that impulses originating from the SA node are transmitted throughout the atria via anterior, middle and posterior pathways (Figure 1), named Bachmans, Wenkebachs and Thorels bundles respectively (Katz, 2006).
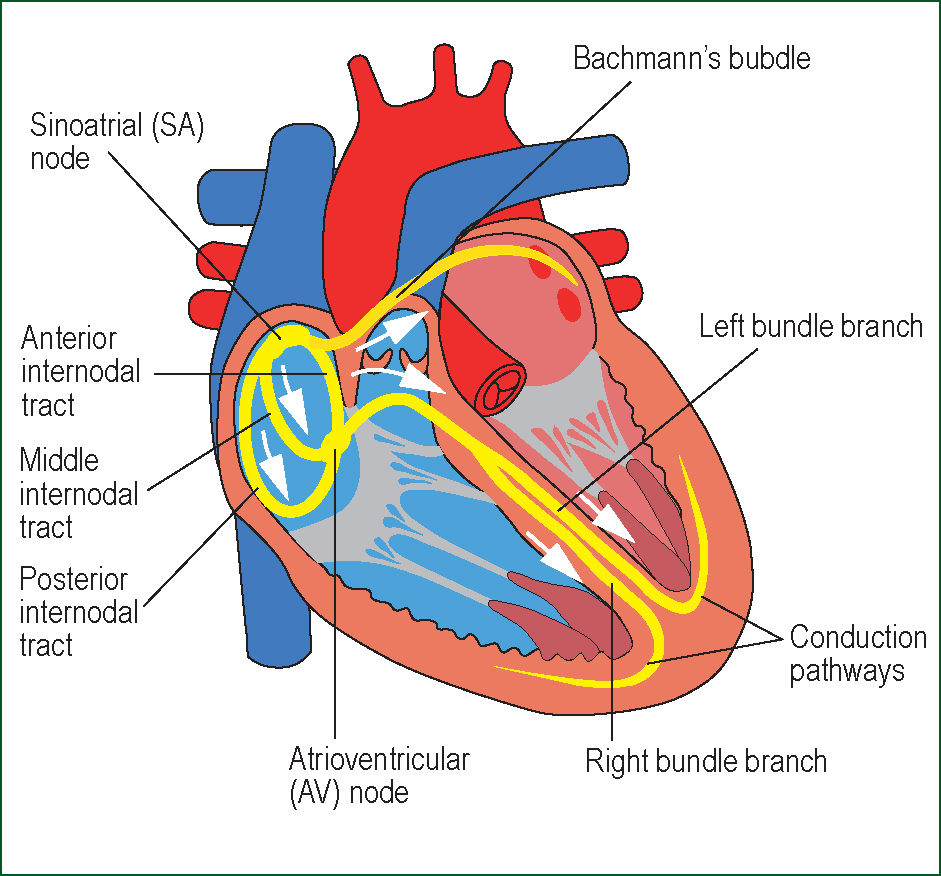
Electrical impulses pass rapidly from the SA node to the atrioventricular (AV) node which is situated on the right atrial wall, directly above the tricuspid valve (McGuire et al, 1992). The AV node consists of a small mass of cells and connective tissue and is normally the only electrical connection across the annulus fibrosus, which insulates the atria from the ventricles. The complexity and small diameter of cells ensures that impulses travelling via the AV node are delayed for approximately 0.1 second before passing into the bundle of His.
The bundle of His conveys electrical impulses through the annulus fibrosus into the upper portion of the interventricular septum, after which the bundle continues along the crest of the main muscular part of the septum. The main bundle then divides into the right (RBB) and left bundle branches (LBB) (Figure 2).

The RBB continues alongside the right interventricular septum and supplies the right ventricle, whilst the LBB further subdivides into the anterior and posterior fascicles (Figure 3), which continue along the left side of the septum and supply the left ventricle. The bundle fibres terminate in an extensive network of Purkinje fibres, designed to distribute electrical impulses to the endocardial myocytes (Levick, 2010).

The artery supplying the SA node frequently originates from the right coronary artery, commonly via the right anterior medial atrial artery, originating at the level of the medial third of the right anterior quadrant of the atrial dome (Katz, 2006). Less commonly, the left coronary artery may supply the SA node via the left anterior medial atrial artery (DiDio et al, 1995). Lopes et al (1998) note that it does not necessarily follow that because the thick walled SA nodal artery crosses the SA node, it provides nourishment. Rather, they postulate that the vessel may have rami that branch in the vicinity of the node, forming an ‘arterial perisinusal network’ that acts as the main source of blood supply (Lopes et al, 1998).
The majority of the blood supply to the interventricular septum is via the penetrating branches of the left anterior descending coronary artery (Figure 4), as posterior septal penetrating branches of the right coronary artery rarely pentrate beyond 15 mm (James, 1965). Blood supply to the AV node is derived from the AV nodal artery, which is a branch of the right coronary artery in 85–90% of cases and a branch of the left circumflex coronary artery in the remaining 10–15% (Katz, 2006). The bundle of His and proximal RBB and LBB frequently receive a dual blood supply, usually via the AV nodal artery and the first septal branch of the left anterior descending coronary artery (Frink and James, 1973).
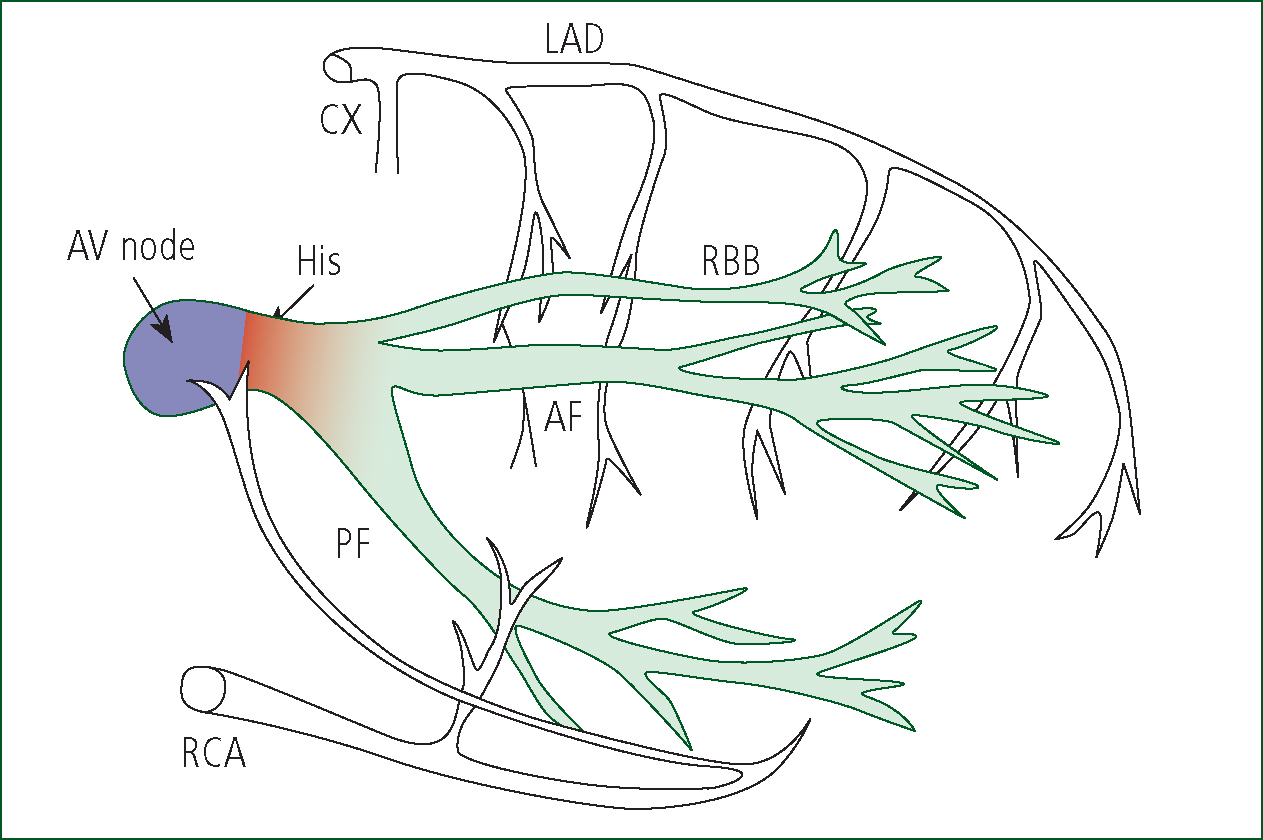
The first portion of the RBB receives twigs from branches of the right coronary artery (Moran and Gunnar, 1975). The second portion of the RBB receives blood from the ramus septi fibrosi and the ramus limbi dextri, a branch of the LAD artery, whilst the final part is supplied via blood vessels from the adjacent myocardium. The first part of the LBB may be supplied solely by the ramus septi fibrosi or may receive a dual supply. The anterior fascicle is mainly supplied by the left anterior descending coronary artery, whilst the posterior fascicle receives a supply via the posterior descending artery of the right coronary artery, supplemented by branches of the distal left circumflex artery (Frink and James, 1973). Although there is considerable potential for collateral circulation to supply the bundle branches, the size and distribution of collateral vessels may prove inadequate in the presence of occlusion of one or more of the coronary arteries providing the dominant supply (Moran and Gunnar, 1975). In approximately 90% of hearts, the posterior descending artery (PDA) branches from the RCA to supply the posterior wall. In the remaining 10% the PDA is a branch of the circumflex artery. These variations are described as right or left dominant respectively (Katz, 2006). The vessels commonly responsible for supplying the various components of the conduction system are summarised below (Table 1).
Component | Blood supply |
---|---|
Sinus |
|
Av node |
|
His bundle |
|
RBB and LBB |
|
Anterior fascicle |
|
Posterior fascicle |
|
Posterior wall |
|
Heart block in AMI
The anatomical site of AMI is important in determining the location and severity of HB (Moran and Gunnar, 1975). The definitions of the various forms of heart block alongside the anatomical and pathophysiological mechanisms associated with each are considered below.
First degree AV block
In first degree HB, every impulse conducts to the ventricle but conduction time is prolonged (Figure 5). Every P wave is followed by a QRS but with a prolonged PR interval (>200 msec) (Ramrakha and Hill, 2012).

First degree HB occurs in less than 15% of patients with AMI admitted to coronary care units and His bundle ECG studies indicate that most patients with first degree HB have intranodal conduction disturbance (Braunwald, 1997). First degree HB is often seen in inferior MI, where occlusion of the RCA compromises blood supply to the AV node (James, 1965). First degree HB in the context of anterior MI indicates extensive damage to the interventricular septum, resulting from occlusion of the LAD artery, and inadequate collateral supply via the AV nodal artery (Connaughton, 2001).
Second degree AV block
Two subtypes of second degree HB have been identified. In type 1, also termed Mobitz 1 or Wenkebach, the PR interval progressively lengthens until a P wave is not conducted and a momentary pause occurs (Figure 6). The PR interval following the dropped beat is the shortest and the RR interval is irregular (Ramrakha and Hill, 2012). QRS width is usually within normal limits (Libby and Braunwald, 2008).

In type 2 or Mobitz 2 there is more than one P wave for every QRS complex (Figure 7), usually in a fixed ratio of 2:1, 3:1 or 4:1 (Ramrakha and Hill, 2012). The PR interval remains constant (Gamon et al, 2007) although QRS prolongation may occur (Libby and Braunwald, 2008).

Type 1 block generally occurs within the AV node and is presumed to be secondary to ischaemic injury following occlusion of the RCA (Libby and Braunwald, 2008). Pathological studies of HB in inferior MI usually demonstrate occlusion of the RCA proximal to the origin of the AV node artery and an absence of major structural damage to the conduction system (Moran and Gunnar, 1975). Type 1 block occurs more frequently in inferior MI, often as a result of increased sensitivity to vagal tone resulting from hypoxia (Feigl et al, 1984). In this context, the block is usually transient and rarely progresses to complete AV dissociation (Gamon et al, 2007). Sutton and Davies (1968) conducted histological studies of 24 hearts from patients suffering inferior MI, in whom the occluded coronary artery had given off the AV nodal artery. The AV node was normal in 22 cases and showed only focal necrosis in two, supporting the presumption of hypoxia producing transient HB (Sutton and Davies, 1968).
Type 1 block in anterior MI frequently involves disease at the level of the His bundle or bundle branches, is associated with widespread necrosis and tends not to resolve readily (Rathore et al, 2001). James (1961) asserts that development of HB in anterior MI must imply additional arterial occlusion of vessels supplying the AV node and posterior wall (James, 1961). This is of particular relevance where combined anterior and posterior infarction occurs (Sutton and Davies, 1968). In cases where the coronary circulation is left dominant, there is clear potential for combined anterior and posterior infarction with associated AV nodal insult and subsequent development of HB. Although the precise mechanisms associated with HB in posterior MI remain unclear, infarction of the AV node, oedema and inflammatory cell infiltration of the node have all been implicated (James, 1962; Paulk and Hurst, 1966). Heightened vagal tone following AMI may also produce varying degrees of HB, particularly in inferior MI (Zimetbaum and Josephson, 2003). The release of substances such as bradykinins and prostaglandins following myocardial ischaemia stimulates ventricular chemoreceptors. These receptors activate the coronary reflex via vagal afferent fibres, producing the Bezold-Jarisch effect, characterised by marked bradycardia and widespread vasodilation (Aaronson et al, 2013). In an animal experiment involving simulated AMI, a comparison of subjects undergoing vagotomy with those not exposed to the procedure demonstrated a significantly lower incidence of arrhythmias in the vagotomy group, thus demonstrating the potential role of parasympathetic tone in arrhythmogenisis (García-Pérez et al, 1980).
In contrast, second degree type 2 HB is more common in anterior MI and is uncommon in inferior MI (Connaughton, 2001). Given that the majority of the interventricular supply is via branches of the LAD coronary artery, second degree HB in the presence of anterior MI is generally associated extensive infarction (Frink and James, 1973). Historically, controversy has existed regarding the precise anatomical location of lesions producing HB in Anterior MI (Sutton and Davies, 1968). Electrophysiological studies utilising His bundle recordings have facilitated more accurate assessment of HB complicating MI (Moran and Gunnar, 1975). Rosen et al (1970) attempted to localise the site of HB by recording His bundle electrograms from MI patients during periods of conduction disturbance. The site of block was determined to be above or below the bundle of His according to the relationship of the His bundle spike to the atrial and ventricular electrograms. Findings in anterior MI were consistent with previous pathological findings. Block occurred below the His bundle recording site, demonstrating disruption to the conduction system distal to the His bundle and providing an explanation for the development of second degree type 2 HB (Rosen et al, 1970).
Third degree AV block
In third degree HB there is complete dissociation between atrial and ventricular electrical activity (Figure 8) (Morris et al, 2008). Both P waves and QRS complexes appear at regular intervals, but are independent of one another (Gamon et al, 2007). Third degree HB may be associated with a normal or widened QRS depending on the aetiology of the block (Libby and Braunwald, 2008).

The bundle of His and proximal bundle branches receive a dual blood supply via the right coronary artery and the septal branch of the LAD artery (Libby and Braunwald, 2008). Development of HB in this context is influenced by both the anatomical location of arterial occlusion and the adequacy of collateral circulation (Moran and Gunnar, 1975). As a result, there is potential for third degree HB to occur in either anterior or inferior MI (Libby and Braunwald, 2008).
Complete HB in inferior MI usually results from an intranodal or supranodal lesion, although high level His bundle block cannot be discounted (Rosen et al, 1970; Bilbao et al, 1987). The potential for a widened QRS and complete heart block in the context of inferior MI further supports the concept of a dual supply to the septum (Frink and James, 1973). In these cases, the left anterior descending artery may have been diseased prior to the development of inferior MI, thus rendering collateral supply via the posterior descending artery more critical than it otherwise might have been. When posterior descending blood flow is reduced following inferior MI, damage to the bundle branches with associated widened QRS may result because the LAD artery is already diseased and is therefore dependent on the posterior descending artery for collateral flow (Moran and Gunnar, 1975). Pathological studies in inferior MI have usually demonstrated occlusion of the RCA proximal to the take-off of the AV nodal artery, without significant damage to the conduction system (Rosen et al, 1970). These findings explain the often transient nature of third degree HB in inferior MI, the potential for normal QRS complexes, and the frequent progression of first and second degree type 1 block to complete heart block in the presence of worsening ischaemia (Libby and Braunwald, 2008).
Development of third degree HB in anterior MI often occurs suddenly within 12–24 hours post infarction, although it is frequently preceded by second degree HB type 2. In contrast to inferior MI, the rate is usually below 40 bpm and is associated with wide QRS complexes (Libby and Braunwald, 2008). Pathological studies in anterior MI have usually identified extensive septal infarction with necrosis involving both bundle branches (Sutton and Davies, 1968; Rosen et al, 1970). James (1962) attributes complete heart block in anteroseptal infarction to AV nodal insult secondary to associated occlusion of the RCA supplying the AV nodal artery (James, 1962). Other investigators have found that the AV node and His bundle were spared and attribute complete heart block in anteroseptal MI to direct involvement of the bundle branches (Blondeau et al, 1967). Although AV nodal dysfunction cannot be excluded, electrocardiographic evidence from continuous monitoring of 24 patients experiencing complete heart block in anterior MI demonstrated a clear relationship between bundle branch block and the development of complete heart block. The authors conclude that consistent evidence of bundle branch block in patients with complete heart block in anteroseptal MI implies that the site of block is at the level of the bundle branches, rather than the AV node and His bundle (Stock and Macken, 1968).
Bundle branch block
Left bundle branch block is associated with broad, notched R waves in Lead 1 and V6, with absent Q waves and a QRS >120 msec (Figure 9) (Ramrakha and Hill, 2012).
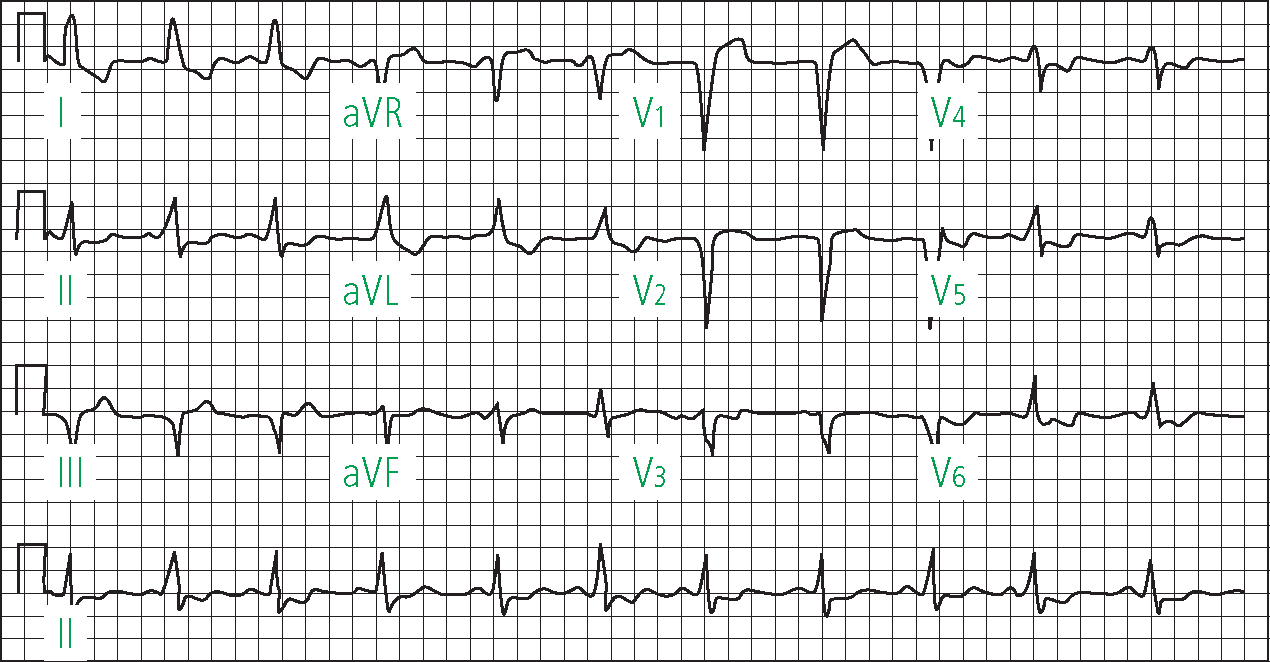
Block of the left anterior or posterior fascicle results in hemiblock. Left anterior hemiblock is characterised by left axis deviation, whilst left posterior hemiblock is associated with right axis deviation (Morris et al, 2003). ECG changes in right bundle branch block include an RSR pattern in V1 and a deep S wave in 1 and V6 (Figure 10). A QRS duration <120 msec in association with the ECG pattern described above is regarded as incomplete right bundle branch block (Ramrakha and Hill, 2012).
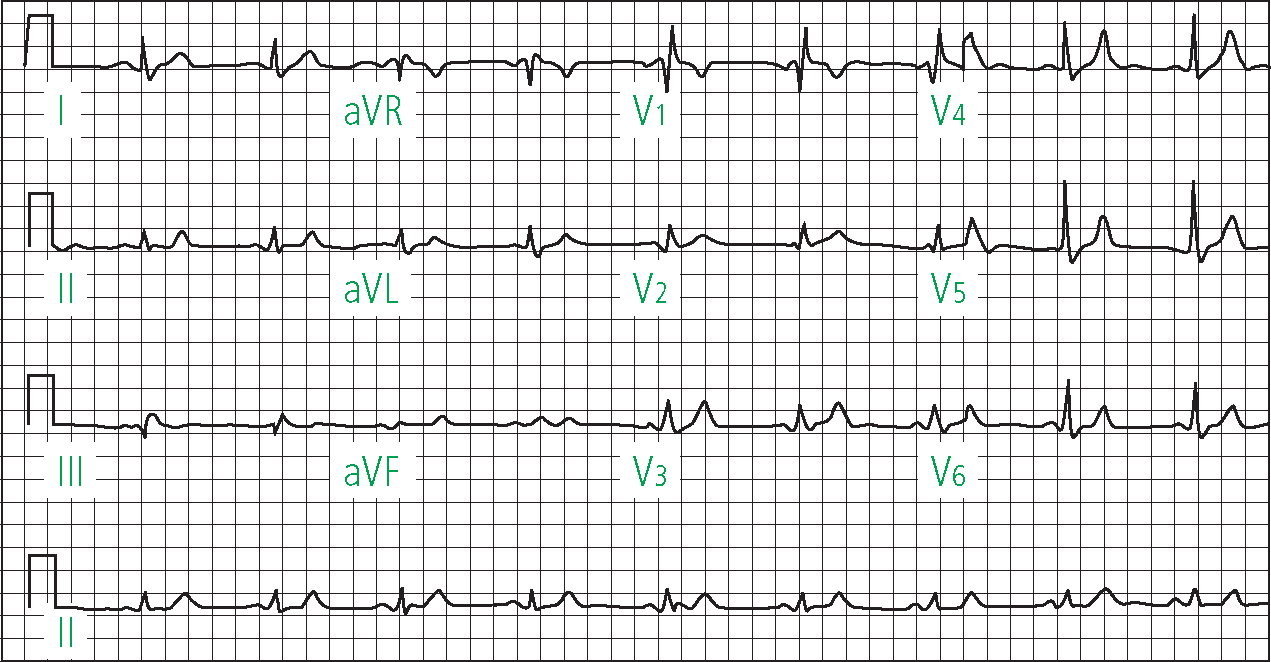
It is prudent to note that not all bundle branch blocks can be regarded as infarct related complications, given that almost half are present at the time of the first ECG and may therefore represent pre-existing conduction disease (Libby and Braunwald, 2008). Transient BBB may also occur in MI, and may be due to reversible ischaemia rather than structural damage (Sutton and Davies, 1968).
The proximal RBB receives a dual blood supply via the first septal branch of the LAD artery and the AV nodal artery. The remainder of the RBB receives branches from the LAD artery and vessels from the adjacent myocardium (Frink and James, 1973). As a result, RBBB is often associated with LAD arterial occlusion resulting from anterospetal infarction (Libby and Braunwald, 2008). In a review of 480 patients with AMI, 18 experienced isolated RBBB. Postmortem findings confirmed that the majority (73%) of patients had suffered RBBB secondary to LAD artery occlusion. Inferior infarction was observed in five patients, with postmortem evidence of fresh RCA occlusion in three and concomitant left coronary artery disease and septal infarction in four (Scheinman and Brenman, 1972). The authors consider pre-existing LAD artery disease with subsequent occlusion of collateral supply via branches of the RCA as the most likely explanation for RBBB in inferior AMI. Alternatively, given the supply of the proximal RBB via the AV nodal branch of the RCA, a small lesion in the vicinity of the RBB may be responsible for isolated RBBB in inferior AMI (Blondeau et al, 1967).
Left anterior hemiblock (LAH) occurs in 3–5% of cases of AMI and is unlikely to progress to complete AV block (Braunwald, 1997). The left anterior fascicle is longer and thinner than the posterior division and does not enjoy the benefits of a dual blood supply, being largely dependent upon the perforating branches of the LAD artery. It is therefore more vulnerable to ischaemia and necrosis, most commonly resulting from LAD artery occlusion in anterior AMI (Kourtesis et al, 1976). Reasons for the development of LAH in inferior AMI are less clear, although pre-existing left coronary artery disease may produce hemiblocks if collaterals from the RCA are interrupted (Scheinman and Brenman, 1972).
Left posterior hemiblock (LPH) is seen in only 1–2% of AMIs (Libby and Braunwald, 2008). The posterior division is short and wide, located in the inflow tract of the left ventricle, which is a less turbulent region than the outflow tract, and has a dual blood supply via the LAD and posterior descending coronary arteries. As a result, it is the least vulnerable segment of the conduction system (Elizari et al, 2007). In cases where isolated LPH is seen, occlusion of the LAD septal perforator arteries secondary to anterior MI is the most common aetiology.
Where LBBB occurs in the context of AMI, the infarct is usually, though not exclusively, anterior, and is associated with significant mortality (Morris et al, 2008). In a postmortem review of eight cases of LBBB, Lev et al (1974) found evidence of myocardial and LBB ischaemia in all. Five cases demonstrated three vessel narrowing or occlusion, whilst only one case demonstrated occlusion confined to the LAD artery. Although more common in anterior than inferior AMI, the key precursor to LBBB is septal necrosis associated with widespread vessel narrowing or occlusion resulting in extensive infarction (Hindman et al, 1978).
Bifasicular block is the combination of RBBB and either left anterior or posterior hemiblock (Morris et al, 2008). RBBB with LAH occurs more commonly due to the relative anatomical vulnerability of the anterior fascicle (Elizari et al, 2007). Incomplete trifasicular block is present where first degree heart block develops in conjunction with a bifasicular block, and may progress to complete heart block (Morris et al, 2008). These defects are more frequently associated with anterior MI (Libby and Braunwald, 2008). The majority of patients with RBBB and LAH will have occlusion of the LAD descending artery with extensive anteroseptal infarction. The comparatively rare occurrence of RBBB and LPH associated with LAD artery occlusion may imply that in some cases the LAD artery constitutes the major blood supply for both fascicles of the LBB (Scheinman and Brenman, 1972). The development of RBBB and LAH in the presence of inferior MI and RCA occlusion further emphasises the anatomical variability in blood supply to the bundle branches (Frink and James, 1973).
Conclusions
Despite increasing use of primary percutaneous coronary intervention (PPCI) for patients suffering AMI and direct transfer by paramedics to heart attack centres in many parts of the UK, the presence of HB remains a marker for comparatively poorer outcomes (Gang et al, 2012; Vivas et al, 2010). This may be influenced by a range of factors, including pre-existing vessel disease, anatomical variation, infarct size and vagal responses (Frink et al, 1973; Katz, 1992; Aarronson and Ward 2004). Although many histological studies investigating anatomical and physiological mechanisms associated with HB in MI originate in the pre-thrombolytic and pre-PPCI eras, evidence from this work remains important in determining contemporary treatment strategies, predicting sequelae and identifying the extent of myocardial insult (Fowler, 2002). Certain forms of heart block are classically associated with infarction of specific anatomical territories. However, the pre-morbid state of the patient coupled with anatomical variations in the coronary circulation highlight the potential for HB to occur as a complication of any AMI.