There are around 30 000 cases of out-of-hospital cardiac arrest (OHCA) resuscitation in the UK each year (Hawkes et al, 2017). Resuscitation of patients in OHCA involves a combination of chest compressions, ventilation, defibrillation and drug administration. As part of advanced life support, the Resuscitation Council UK (RCUK) states that ventilations should be conducted at a rate of 10 per minute, and the RCUK's post-resuscitation care guidelines recommend varying the ventilation rates and volume to target a normal arterial partial pressure of carbon dioxide (PaCO2) of 4.5–6.0 kPa (Perkins et al, 2021a).
Typically, cardiac arrest results in combined respiratory and metabolic acidosis as pulmonary gas exchange ceases and cellular metabolism becomes anaerobic, resulting in cellular hypoxia and hypercapnia (Robba et al, 2020). This combination can reduce the effectiveness of drugs given to restart the heart, reduce the oxygen-carrying capacity of haemoglobin owing to its displacement by carbon dioxide, reduce myocardial contractility and cause myocardial irritability, therefore reducing the likelihood of sustaining a return of spontaneous circulation (ROSC) (Association of Ambulance Chief Executives, 2022).
Despite the RCUK guidelines, many clinicians still ventilate outside its recommendations, some through a lack of awareness of the rate and tidal volumes at which they are delivering ventilations, while others may opt to perform hyperventilation during resuscitation as ventilation with higher rates or volumes can decrease PaCO2 and lead to the pH being normalised more quickly (Skrifvars et al, 2019). However, a swine study by Aufderheide et al (2004) showed hyperventilation could lead to barotrauma, hypotension, diminished pulmonary blood flow and reduced cardiac output.
The aim of this review is to describe the incidence, causes and outcomes of hyperventilation provided by emergency medical service clinicians during the management of an OHCA.
Methods
This review followed a focused literature search of three databases—CINAHL, MEDLINE and ScienceDirect—from 1 January 2000 to 27 April 2022. Only human, adult, prehospital studies were included. Papers were selected after the year 2000 to ensure relevance to current and progressing paramedic practice (Table 1; Table 2).
Patient group | Intervention | Comparison | Outcome |
---|---|---|---|
Out of hospital |
Resuscitation |
Normo-ventilation | Return of spontaneous circulation (ROSC) |
Phrase searching: words within groups combined with AND/NOT/OR;
truncation;
EMS: emergency medical services
Inclusion criteria | Exclusion criteria |
---|---|
Out-of-hospital medical cardiac arrest |
Traumatic cardiac arrest |
The lead reviewer (BK) completed the initial search, applied the eligibility criteria and screened the titles and abstracts. A second reviewer (SH) reviewed 10% of the full-text articles and, where necessary, a third reviewer (GM) acted as a mediator.
After abstracting the relevant data, a Critical Appraisal Skills Programme (CASP) (2023) evaluation was used to appraise the evidence. A CASP tool that was appropriate and relevant to each study design was used to identify quality of evidence and risks of bias.
Findings
The search strategy identified 326 potentially relevant studies, of which 272 were excluded after titles and abstracts were screened. Forty-one were excluded after the full text was reviewed. Thirteen studies detailing hyperventilation during adult OHCA were then included in the review (Figure 1).
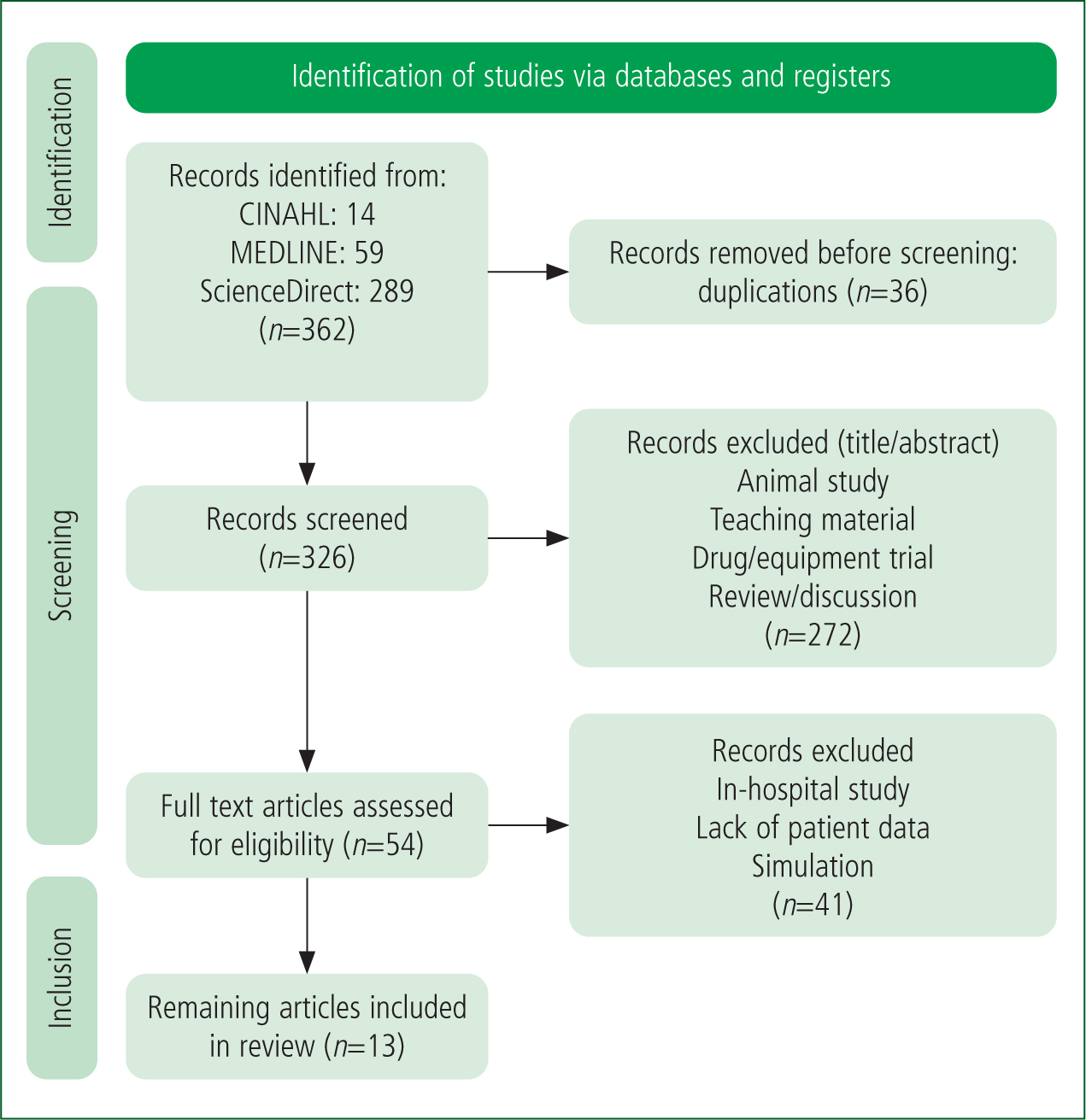
The 13 papers included a total of 2575 cases of resuscitation in six countries, with papers published between 2004 to 2021. No human randomised control trials were identified. CASP (2023) evaluation showed the papers to be of a largely good quality design, with moderate risks of bias identified and results that could be applied to a wider population. No papers were excluded because of quality alone. It was noted that none of the papers included were designed to investigate the occurrence and patient outcomes of hyperventilation specifically; conclusions have been inferred from evaluations into the quality of cardiopulmonary resuscitation (CPR), including the delivery of ventilations.
Because local guidance and progressive updates in evidence-based recommendations differed, ventilation rates and volume varied over the course of the studies included in this review; nonetheless, hyperventilation, regardless of guideline followed, was still commonly reported in OHCA (Table 3; Figure 2).
Studies organised by ascending average ventilation rate | |||||
---|---|---|---|---|---|
Study (year) | Country | Number of participants | Average ventilation rate (per min) | Error range (ventilation) | Outcome: return of spontaneous circulation |
Sanson et al (2019) | Italy | 285 | 7.7 | 3.9 | 28.2% |
Sheak et al (2015) | United States | 356 | 8.0 | 1.5 | 27.0% |
Becker et al (2018) | United States | 126 | 8.3 | 2.6 | 22.6% |
Olasveengan et al (2009) | Norway | 917 | 10.0 | 4.0 | 38.0% |
Murphy et al (2016) | United States | 230 | 10.4 | 3.4 | 30.0% |
Wik et al (2005) | Norway/England/Sweden | 176 | 11.0 | 4.7 | 35.0% |
Van Den Daele et al (2021) | Belgium | 10 | 12.0 | 1.8 | 33.0% |
Olasveengan et al (2007) | Norway | 121 | 13.0 | 4.0 | 31.0% |
Olasveengan et al (2008) | Norway | 75 | 13.0 | 4.0 | 53.0% |
Vissers et al (2019) | Belgium | 337 | 15.3 | 6.5 | 41.5% |
Duchatelet et al (2018) | Belgium | 25 | 18.0 | 2.6 | 32.0% |
Maertens et al (2013) | Belgium | 43 | 20.0 | 6.8 | 56.0% |
Aufderheide et al (2004) | United States | 13 | 30.0 | 3.2 | 0.0% |
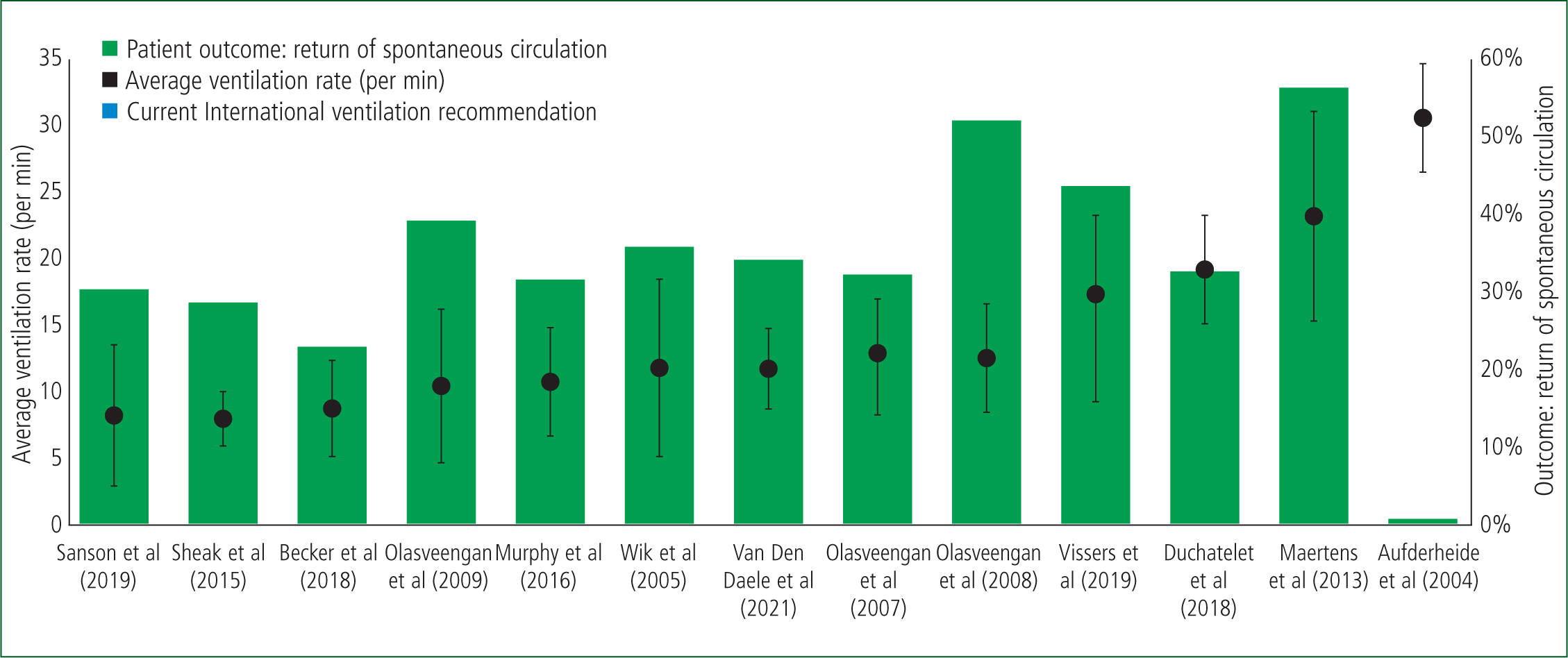
Maertens et al (2013) reported cardiac arrest patients were manually ventilated at an average rate that was twice that in the recommended guideline; further to this, Duchatelet et al (2018) reported ventilation rates well exceeding recommended guidelines, averaging 18 per minute, and found hyperventilation through excessive tidal volumes, with 75% of patients being ventilated with a tidal volume greater than 600 ml. Finally, Vissers et al (2019) showed that 85.2% of patients received more than 10 ventilations per minute but found no significant differences in patient outcomes regardless of ventilation rate.
Discussion
The dangers of hyperventilation were prominently highlighted by Aufderheide et al (2004). Their paper reported ventilation rates in excess of 30 per minute, more than double the 12–15 per minute recommended in the American Heart Association (AHA) guidelines at the time of their study (AHA and International Liaison Committee on Resuscitation, 2000); this was associated with very poor patient outcomes, with no patients experiencing ROSC. Concerns around hyperventilation were so great that investigators ceased their study to ensure clinicians were retrained to deliver ventilations at a rate of 12 per minute.
Nearly 20 years later, international guidelines agree that ventilations should be delivered at a rate of 8–10 per minute with a tidal volume of 6-8 ml/kg; on a practical level, this should be enough to produce a visible chest rise (Perkins et al, 2021b).
Despite hyperventilation still being a common occurrence during OHCA, increasing compliance with guidelines was reported by Olasveengen et al (2007), with a decrease in average ventilation rates from 16 per minute to 12 per minute over a 3–year period from 2003 to 2006. Van Den Daele et al (2021) found low levels of hyperventilation, with an average of 12 per minute with only 20% of tidal volumes being in excess of 600 ml, and Becker et al (2018) showed good compliance, with clinicians delivering manual ventilations of 8.2 per minute and 8.5 per minute through endotracheal tube and supraglottic airway devices respectively.
Despite a focus on educating clinicians on current resuscitation practice, ventilations are still being delivered outside recommended rates and tidal volumes. Van Den Daele (2021) notes that, even for trained and experienced clinicians, OHCA resuscitation can be a stressful event. This was previously noted by Maertens et al (2013), who proposed that hyperventilation may result from stress in team members, and confounded by poor on-scene clinical leadership failing to correct rates and volumes. McInnes et al (2012) showed that 94% of team leaders noted excessive ventilation rates during cardiac arrest resuscitation but failed to act on it. Maertens et al (2013) also suggested there was a need for further education with a focus upon immersive training to simulate the stresses associated with performing CPR in an uncontrolled setting which may better reflect clinician performance during a real-life resuscitation than a neutral or traditional simulated environment.
Ventilation feedback devices have been trialled to improve compliance with guidelines. In a simulated study, Charlton et al (2021) reported that, without ventilation feedback, clinicians delivered only 51% of ventilations at the correct rate, and showed hyperventilation with excessive tidal volumes during 57% of manual ventilations. However, when using a ventilation feedback device, their compliance greatly improved, with the correct rate and tidal volume delivered for 94% of ventilations.
Even when ventilation feedback devices are used, clinicians do not always follow the feedback, leading to short episodes of hyperventilation occurring; practitioners have said this was usually because they were being distracted by other on-scene elements or because there were planned pauses in ventilations when they were concentrating on other clinical interventions (Wik et al, 2005).
Aufderheide et al's (2004) human observational study was supplemented by a porcine study that investigated the effects of excessive ventilation rates in pigs, finding a significant reduction in coronary perfusion pressures leading to an increased likelihood of death. This study has provided the basis of International Liaison Committee on Resuscitation recommendations for avoiding hyperventilation. Since then, however, these concerns have been refuted by Gazmuri et al (2012), who found increasing respiratory rate and tidal volume up to a minute-volume 10-fold higher than the then recommended guidelines had no adverse haemodynamic effects during CPR. This was supported by Vissers et al (2019), who reported no significant differences in ROSC, survival to hospital discharge or 1-year survival in patients receiving ventilations at a rate of >10 per minute compared to those who received ventilations at ≤10 per minute.
Internationally, OHCA survival rates have been improving, with 1-year survival rates increasing from 8.0% in 2000–2009 to 13.3% in 2010–2019. Reported ROSC rates globally show great variety depending upon location. Oceania displays the highest incidence of ROSC at 38.6%, followed by Europe at 36.7%, while North America and Asia report ROSC rates of 24.3% and 22.1% respectively (Yan et al, 2020). Within the studies included in this review, patient outcomes also showed great variety.
The studies in this review share some common limitations, primarily the reported use of mean ventilation rates during cardiac arrest resuscitation. During a cardiac arrest, ventilation rates will naturally vary dependent upon the operator, airway device and chest compression-to-ventilation ratio used. Before an advanced airway device is inserted, clinicians are encouraged to perform CPR at a synchronised ratio of 30:2 then, once a supraglottic airway or endotracheal tube has been placed, continuous ventilations and chest compressions can then be adopted (Olasveengen et al, 2021).
Synchronised versus asynchronous ventilations and chest compressions were investigated by Sanson et al (2019), who found that, if the guidelines and ratio were followed correctly, a maximum of 4–6 ventilations per minute should be given; however, they found a mean of seven ventilations per minute were consistently delivered. Sanson et al (2019) went on to report that ventilation rate was independently related to a patient's outcome, observing that every additional breath per minute led to a 3.8 increase in the odds of a good neurological outcome.
Previously, Wik et al (2005) had found the mean ventilation rate was lower during the first 5 minutes of resuscitation than during later resuscitation, surmising this may result from changing compression ratios and the time spent performing other clinical interventions, such as gaining intravenous or intraosseous access, or placing advanced airway devices.
Many of these studies used trans-thoracic impedance (TTI) to determine the ventilation rate through changes induced by chest wall movement noted by the defibrillation pads. TTI signals can be compromised through artefacts from different sources, including chest compressions (Edelson et al, 2010). Waveform capnography can also be used to monitor ventilation during CPR. Both methods use algorithms to calculate the ventilation rate and both can be compromised through interference and artefacts. There does not appear to be a consensus on superiority in determining ventilation rate between TTI or waveform capnography.
Conclusion
Despite ventilations being consistently delivered at a rate and tidal volume higher than is internationally recommended, there are no discernible clinically significant differences in patient outcomes between ventilation rates of ≤10 per minute and to >10 per minute. Currently available human data do not produce sufficient evidence to favour any ventilation strategy. However, a harmful limit will exist; the upper cut-off limit for ventilations during out-of-hospital management of an adult medical cardiac arrest is not known.
This review found no human randomised control studies examining how out-of-hospital delivery of ventilation rates, tidal volume or pressure by emergency medical services clinicians affects patient outcomes following an OHCA and this warrants further research.
Ventilation feedback devices have an important role and can be used to guide clinicians in delivering ventilations at the recommended rate and tidal volume. Practitioners should receive regular education to ensure they are up to date on current guidelines and undertake high-fidelity scenarios to simulate real-world stressors.